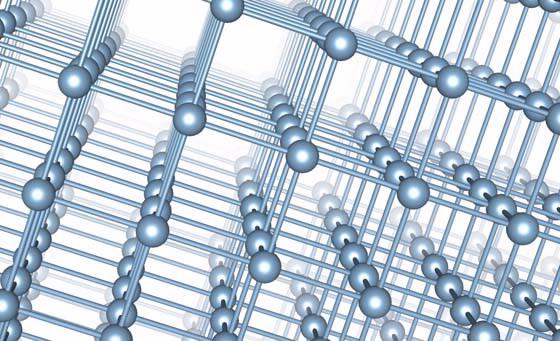
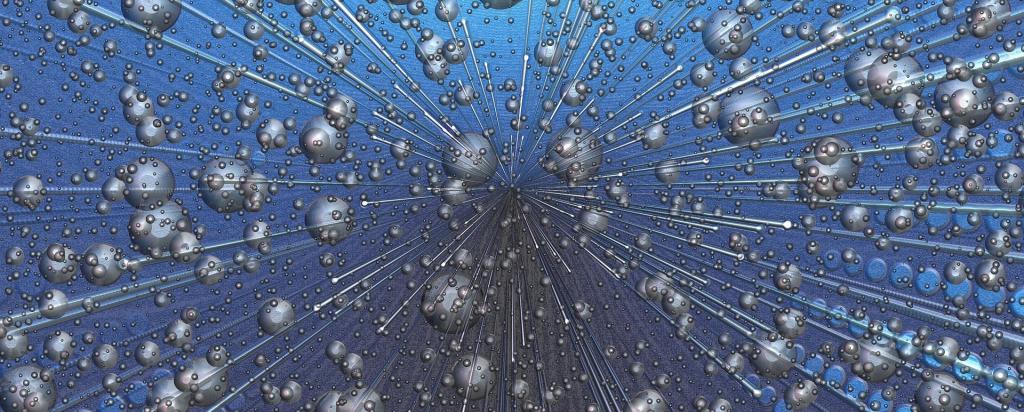
Published on the 1st September 2015 by ANSTO Staff
In a real-life scenario the loss of a coolant, which removes heat (normally water but also sometimes gas or molten salt), from the core of a nuclear reactor can have a significant, damaging impact.
Research using atomic scale computer simulations at ANSTO on accident tolerant nuclear fuels and cladding materials has the potential to design better components that can handle a ‘loss of coolant’ scenario in light water reactors (LWRs).
“Because the applications of this research are already in part being used at ANSTO’s OPAL reactor, we have great confidence in our safety features and we can lead the way in improvements to the design of materials for use in commercial reactor environments,” according to Greg Lumpkin, Programme Leader, Nuclear Materials Modelling and Characterisation Group at ANSTO.
Members of the group are part of an international project undertaken in association with Westinghouse Electric, universities and laboratories in the US, Sweden and Japan to develop safer nuclear fuel materials for use in both research and power-generating reactors.
The group is using quantum mechanical computational models and simulations to predict their behaviour under extreme conditions. They can effectively predict how fuel and cladding material should behave in a reactor environment before experimental testing takes place.
Modelling on the atomic scale provides a mechanistic understanding of a material’s behaviour. If you know what happens in a very limited range of conditions, you can predict what will happen in more extreme conditions, such as a loss of coolant.
The real safety of a nuclear fuel system under conditions such as extreme temperature, pressure and radiation can be evaluated by combining an understanding of the fundamental structural properties of fuel and core vessels with experimental data.
“Modelling based on density functional theory lets us know what is happening with the chemistry of the materials. We get a very accurate representation of how the materials react, change properties or fail under extreme conditions,” explained former group member Simon Middleburgh.
Accident tolerant fuels, such as high density fuels, can be used in both current and next generation LWRs that use pressurised water or boiling water.
Uranium silicide is a candidate safe fuel system that is used in ANSTO’s OPAL reactor. A loss of coolant event at the OPAL reactor is essentially avoided because the core is contained in a 12.8 metre open pool of water that is replenished by gravity and doesn’t rely on power. However, the lessons learned by operating silicide fuels in this extremely controlled system will allow advances in the development of fuel exposed to the more efficient but harsher environments that exist within a commercial power reactor.
The international group hopes to design a new fuel that can withstand a loss of coolant accident indefinitely. In this situation, nuclear materials would experience constant temperatures greater than 1500o Celsius for many days.
“Modelling is a cheap, safe, quick and relatively easy method of analysis. We can make educated guesses about the behaviour of many new materials and pick the best candidates before experimentally verifying their behaviour,” said Middleburgh.
“Modelling is a cheap, safe, quick and relatively easy method of analysis. We can make educated guesses about the behaviour of many new materials and pick the best candidates before experimentally verifying their behaviour,” said Middleburgh.
In addition to the work on fuels, ANSTO-based research has focused on the best materials to encase nuclear fuel. The cladding is the first barrier between the fuel and the external environment. You can increase the level of safety in the event of an accident by preventing decomposition and preserving the structural integrity of nuclear materials.
High entropy alloys are a new class of materials that include equal amounts of a combination of metals which gives rise to superior strength, hardness and ductility. Current work attempts to tailor these alloys for radiation, corrosion and oxidation resistance has shown promising results.
Daniel King, a PhD student with an undergraduate degree in nanotechnology who is considered an expert atomic modeller of high entropy alloys, has been evaluating these materials, at an atomistic level, for potential use in the next generation of nuclear reactors.
“We have the physics to develop these reactors but we don’t have the materials to engineer them,” said King.
Using ion bombardment from the ANTARES accelerator at ANSTO (under the supervision of Mihail Ionescu), he can simulate a metallic alloy’s response to extreme levels of radiation and compare these results to the computational predictions to assess the overall radiation tolerance. The ion accelerators can be used to simulate interaction of fission products with nuclear materials outside nuclear reactors, offering significant advantages.
When an energetic ion beam from an accelerator hits a sample it will interact with the target atoms via a direct interaction with the nuclei and with the electron clouds. For light ion beams, an additional interaction mechanism is possible via nuclear reactions, similar to the neutrons in a reactor. The interaction of swift heavy ions with nuclei and electron clouds of target materials result in the deposition of kinetic energy which can lead to atomic displacements and therefore defects.
It is theorised that a high entropy alloy will have an enhanced “self-healing” effect to maintain structural integrity and are expected to be a significant improvement over other options such as SiC-SiC composites that have far lower radiation tolerances and thermal conductivities.
The modelling of this behaviour under irradiation is currently one of the only methods for accurately predicting radiation tolerance within materials. Although the raw data is mathematical, there are programs that depict useful dynamic three-dimensional images of the atoms.
King says they are assessing the suitability of alloys which have not previously been considered for use in reactors. Existing cladding materials such as Zircaloy-2 and 4 are comprised of one primary element, zirconium. One of the new high entropy alloys, NbTiVZr, is being evaluated using atomic scale simulations.