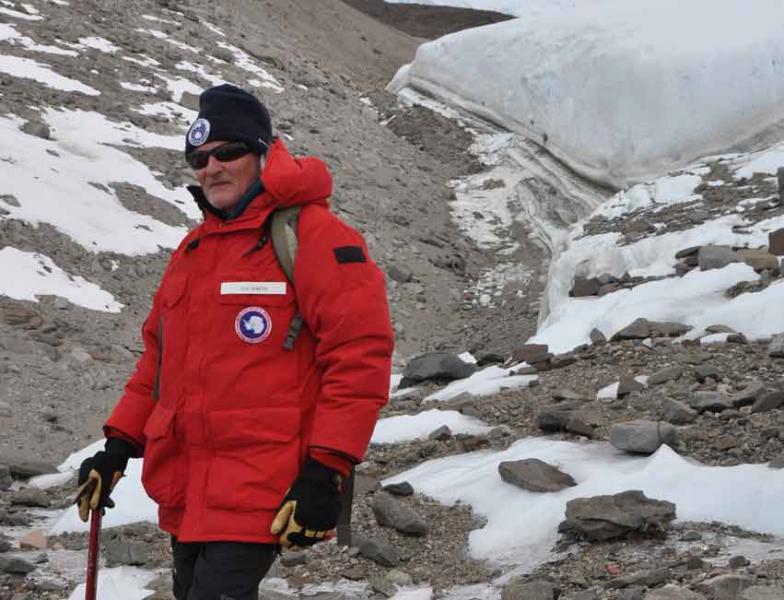
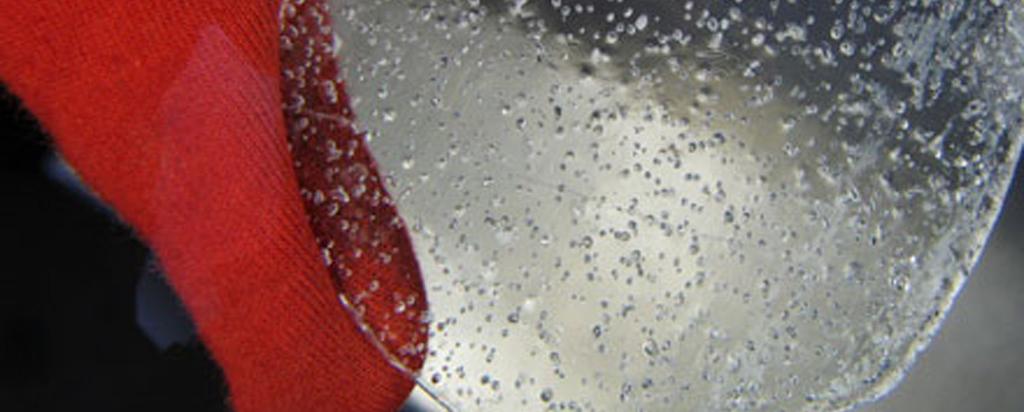
Published on the 28th November 2018 by ANSTO Staff
This summer, an international team is assembling at Australia’s Casey Station in Antarctica to undertake climate research. The team of 10 will travel 125 km over snow to a deep-field camp near the summit of nearby Law Dome and will be there for almost three months in a mobile laboratory. The team comprises scientists, technicians, students and support personnel from the University of Rochester (USA), Ice Drilling Design and Operation (IDDO, Wisconsin, USA), CSIRO, Australian Antarctic Division (AAD) and ANSTO.
The expedition, which will include Dr Andrew Smith, will even have its own science film maker. This expedition is the culmination of over four year’s planning.
The project seeks to learn more about a very important component of the atmosphere – the hydroxyl radical, with the chemical symbol OH – a molecule comprised of one atom of oxygen and one atom of hydrogen. What makes OH special is that it has an unbound electron in its outermost orbital and that makes it chemically reactive.
In fact it makes it so reactive that OH has an average lifetime of around one second before it reacts with something and is consumed. It plays a fundamental role in atmospheric chemistry because it reacts with and removes trace gases from the atmosphere, including greenhouse gases and ozone-depleting gases. This is what makes it so important and why it is often called ‘the detergent of the atmosphere’.
However, with such a short lifetime it is impossible to measure OH directly in the atmosphere. Instead, its concentration must be inferred by seeing its effect upon other gases. Until recently a man-made compound, methyl chloroform, was used as a ‘proxy’ for OH.
This was possible because the amount of methyl chloroform produced was very well known, so measurement of the amount remaining in the atmosphere told us how much must have been removed by OH and so how much OH there must have been to react with it. However, methyl chloroform is an ozone depleting substance and was banned by the Montreal Protocol in 1989; concentrations have now dropped to the point where these measurements are no longer useful. Furthermore, production only commenced in about 1978 and prior to this date we have no knowledge of atmospheric OH levels.
This is where radiocarbon or 14C comes into the story. 14C is produced in the atmosphere by primary cosmic rays, or more correctly by secondary neutrons produced as the very energetic cosmic rays collide and lose their energy.
These neutrons are captured by the very abundant nitrogen (14N) which is transmuted into 14C and this in turn is quickly oxidised into radiocarbon monoxide – 14CO. Changes in the atmospheric concentration of 14CO not explained by changes in production rates are mainly due to changes in OH, its main ‘sink’.
In collaboration with the University of Rochester, ANSTO is measuring 14CO in the current atmosphere in samples taken at the Mauna Loa Observatory in Hawaii. This project continues the contemporary OH record, using much smaller air samples than was previously possible. ANSTO’s capability for precisely measuring 14C in miniscule carbon samples makes this approach possible.
But what of OH concentrations prior to 1978? We believe that we can use the same technique, but by sampling air that has been trapped as bubbles in ice cores to access these past times. As snow falls in polar regions it doesn’t melt and is slowly compacted by the weight of overlying snow.
The air in between the snowflakes gradually becomes trapped as bubbles and then moves with the ice. In a region where the ice stratigraphy is undisturbed, drilling into the ice sheet yields older and older samples of the Earth’s atmosphere. This is how we know of the significant increase in greenhouse gases brought about by mankind through industrialisation and agriculture.
But there are some rather formidable challenges to overcome before using 14CO from ice core bubble air as a proxy for OH. Firstly, there is not very much carbon monoxide in air to begin with… it varies seasonally, between about 40 and 80 parts per billion (ppb). So, comparatively large ice samples must be collected just to get enough carbon monoxide. In our case, we needs to melt approximately 300 kg of old ice to liberate enough air for the experiment (~ 38 L).
With such low concentrations we must be absolutely scrupulous to avoid introducing any contaminants and the melting vessel itself has been specially designed to minimise this.
Another formidable problem is that 14C is produced in the ice itself, again by secondary particles produced by cosmic rays. These particles (neutrons, negative muons and fast muons) have different properties and penetrate the ice to differing degrees. We have specifically chosen a site where the snow accumulation is very high, at least from an Antarctic perspective.
This will help in shielding the ice from in situ 14C production, making the experiment feasible. Furthermore, the high accumulation rate results in a record that is tightly resolved in time. It will mean, however, that we spend a lot of time and energy just keeping our camp from being buried!
On this expedition we will have three ice drills: nominally 3”, 4” and 9½”. The 3” drill will be used to penetrate the accumulating snow (the ‘firn’), allowing us to sample the air before it is trapped as bubbles.
The 9½” drill will be used to take large samples in the critical region of ‘bubble-close of’ at a depth of about 80 m at this site. The 4” drill will be used to drill three parallel boreholes below this depth and to a maximum depth of approximately 250 m, taking us back to an air age around 1850. The air liberated from these cores will provide the radiocarbon monoxide for our study.
After the air has been collected in the field, it will be shipped to the University of Rochester for the separation of CO and CH4 (methane). Once again we have to be careful because further 14C will be produced in the air cylinders themselves as they are transported by plane; we allow for this by shipping cylinders depleted of CO along with the actual samples cylinders.
Once separated, the CO and CH4 are each oxidised to CO2 and placed in glass tubes for the journey back South to ANSTO.
At ANSTO, we will convert that CO2 into graphite, the solid form of carbon we use as a target in our particle accelerators.
The 10 million volt (MV) tandem accelerator Antares will then count the 14C atoms in the target, one by one, as they enter the nuclear physics ionisation detector at the end of the beam line.
We expect to end up with samples of just 20 millionths of a gram of carbon, containing the handful of 14C atoms from the 14CO extracted from the ice cores. These will be measured by accelerator mass spectrometry (AMS) in ANSTO’s Centre for Accelerator Science (CAS) where we can detect just one atom of 14C in amongst 10,000,000,000,000,000 (1016) ‘normal’ carbon atoms. In terms of sizes, this is equivalent to laying a virus across the Earth’s equator.
ANSTO is one of the few laboratories in the world that can make accurate radiocarbon measurements with this sensitivity on such small samples.
Content provided by Dr Andrew Smith
Read the media release
Watch the video on CSRIRO blog