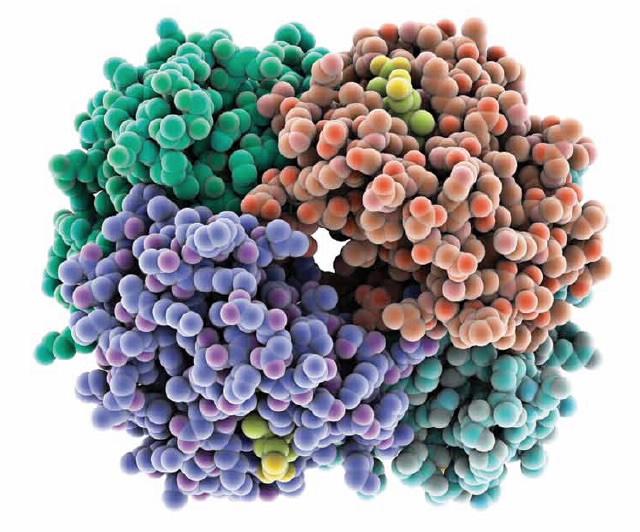
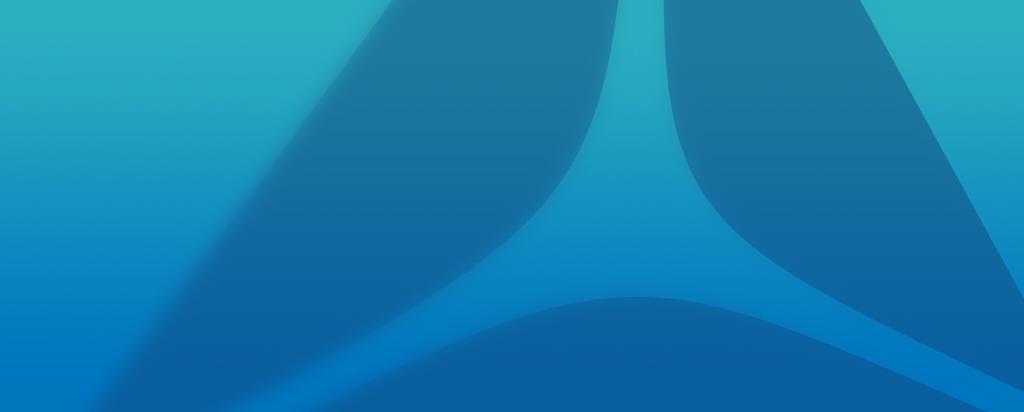
Published on the 15th May 2014 by ANSTO Staff
![]()
Haemoglobin molecules store oxygen in the body, and red blood cells transport this vital storage unit around the body. Haemoglobin is essential for the effective functioning of our bodies, with haemoglobin deficiencies causing diseases such as anemia. It is therefore important that we understand how haemoglobin works and what factors affect its functioning. In this study, researchers look at how haemoglobin molecular operations are optimised based on body temperature by comparing haemoglobin function in different animals. Chris Garvey and researchers from France and Germany have been working to gain a better understanding of the molecular basis for this optimisation which involves a complex interplay between structure and the internal flexibility of the structure. To undertake this study, the researchers selected haemoglobins from species that regulate their body temperature over a range of external conditions, including platypus, domestic chicken and humans, and species that do not have a regulated body temperature, including the salt water crocodile. The researchers discovered that there is a positive correlation between the thermal stability of the environment around these oxygen storage cells (i.e. the protein) and their resilience - shedding light on how haemoglobin may have evolved to carry oxygen best at different body temperatures in different species. Computer simulations were used to examine which part of the haemoglobin molecule from different species was responsible for the change in the overall softness of the protein. Having gained a better understanding of haemoglobin function, the next steps are to examine observations in the context of oxygen affinity, or how well haemoglobin molecules hold on to oxygen. This will be possible with further development of instruments at ANSTO’s OPAL research reactor facilities. IntroductionMolecular biology tools have made it possible to test hypotheses about the functioning of biomolecules by directly manipulating the structure. However, this research followed an older experimental tradition, comparative physiology, where aspects of biological functioning are compared in the context of animals with differing physiology [1]. This traditional method was coupled with the tools of modern soft condensed matter science; computer simulations together with neutron and optical spectroscopy, which measure the momentum and energy changes to neutrons when they interact with a sample, to reveal information about the softness of protein [2]. Haemoglobins were selected from species that regulate their body temperature over a range of external conditions (platypus, domestic chicken and humans) and a species which does not have a regulated body temperature (salt water crocodile). Experimental techniques revealed not only changes in arrangements and internal motions of the molecule within the basic globular shape but also in thermal stability of each haemoglobin molecule. Researchers found a positive correlation between the thermal stability of the environment around these oxygen storage cells (i.e. the protein) and its resilience. HaemoglobinAlthough there is a high degree of similarity between haemoglobin molecules from different species, computer simulations were used to examine which part of the haemoglobin molecule from different species was responsible for the change in the overall softness of the protein. Having gained a better understanding of haemoglobin function, researchers are now looking to examine these observations in the context oxygen affinity, i.e. how well haemoglobin molecules hold on to oxygen, which will be possible with further development of instruments at ANSTO’s OPAL reactor facilities.
Haemoglobin is a polymer of various amino acids (protein) which in its native state is folded into a compact globular shape which includes four separate haemoglobin molecules (Fig. 1). Invertebrates the haemoglobin molecule has been enclosed in a highly specialised cell known as the erythrocyte, or red blood cell. The haemoglobin tetramer, Hb4, is predominantly found inside red blood cells. The red blood cell is a recent evolution; it is present in the circulation of most vertebrates where it carries an extremely concentrated solution of the oxygen storage protein haemoglobin (about 30%) around the body where it is essential for metabolic processes. In its normal functioning in endotherms (animals that metabolically regulate their body temperature, e.g. platypus, domestic chicken and human) it is regulated at a relatively narrow range of temperatures, the body temperature. Ectotherms (e.g. the saltwater crocodile) do not metabolically regulate their body temperature but instead use behavioural strategies. The physiological problem that must be solved by the haemoglobin molecule is that it must store oxygen from the atmosphere in the lungs, and in the peripheral circulation in the small blood vessels found in many sorts of tissue; but it must also release the oxygen so that it may be used for metabolic activity. Oxygen is held in one of four heme groups (Fig. 1). Various factors are already known to influence the binding of O2 to these sites including the binding of other O2’s to the other sites within the tetramer, the presence of different phosphate molecules which are related to cell metabolism [3] and pH. In this study we have examined the haemoglobin from endotherms with a range of body temperatures and an ectotherm in the context of the operating body temperature (Table 1 [4]).
The Measurements The backscattering spectrometers IN13 [5] and SPHERES [6] were used to study the scattering of neutrons, or the change in the path of neutrons as a function of angle as well as the energy change in these neutrons. The information obtained was the average fluctuation of hydrogen around its average position within the Hb4 molecule. For hydrogen atoms on a ridged molecule this value is small. For softer molecules the fluctuation is greater and made as a function of temperature the measurement allows us to measure a mean force elastic constant. Our measurements suggest that that a certain amount of water is necessary to obtain and maintain the internal motions necessary for biological function of haemoglobin, but also at this level for the endotherm the force constant parallels the order of the body temperature, that is to say the platypus has the softest haemoglobin and that the chicken has the stiffest (table 1). The crocodile’s haemoglobin is quite stiff ompared to the other haemoglobins but it must function over a range of temperatures.
Circular dichroism spectroscopy measures the differences in absorption of two different polarisations of light in the ultra-violet/visible regime. This method was used to determine the different folding of the four haemoglobin molecules within the overall globular shape as a function of temperature. Specifically we focussed on the loss of a particular aspect of the structure, the α-helix, using the spectra at the 222 nm (ultraviolet) region, and a temperature at which there is a partial unfolding of the structure. We found that for the endotherms studied the order of the unfolding temperature is the same as the body temperature. These measurements are used to indicate the overall stability of the haemoglobin molecules in its functional structure. Combining results with the computer simulationsWhile the haemoglobins molecules for each species are quite similar, they all fold into the same basic tetrameric globular structure, but they differ subtly by the chemistry along the chain of the haemoglobin. We have used computer simulations to understand which regions of the folded protein are responsible for the changes in the thermal stability and overall softness of the proteins. The initial step was to build a credible three dimensional model of the arrangements of the molecule within the overall globular shape—the precise three dimensional structure is not known for platypus and crocodile haemoglobin. The simulations allowed us to determine which residues are responsible for changes in the force constant for the overall molecules determined by neutron spectroscopy. In terms of the biological function of the haemoglobin molecule in different species we have investigated the rigidity of the environment (protein) around the oxygen storage site. Each Hb4 molecule must function to allow the oxygen to bind and be released according to physiological need. Our further investigations aim to understand these observations in the context of oxygen affinity, but we also speculate that some of the mediators of oxygen affinity may be associated with changes in the internal dynamics of the haemoglobin molecule. Further developments of the instrumentation at the OPAL reactor will allow us to pursue our research at Australian facilities. References
|