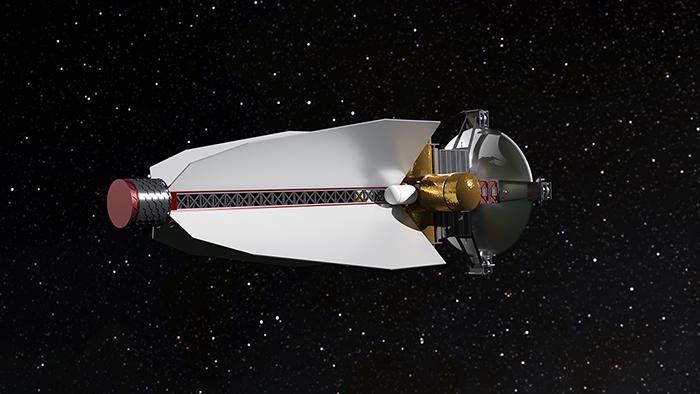
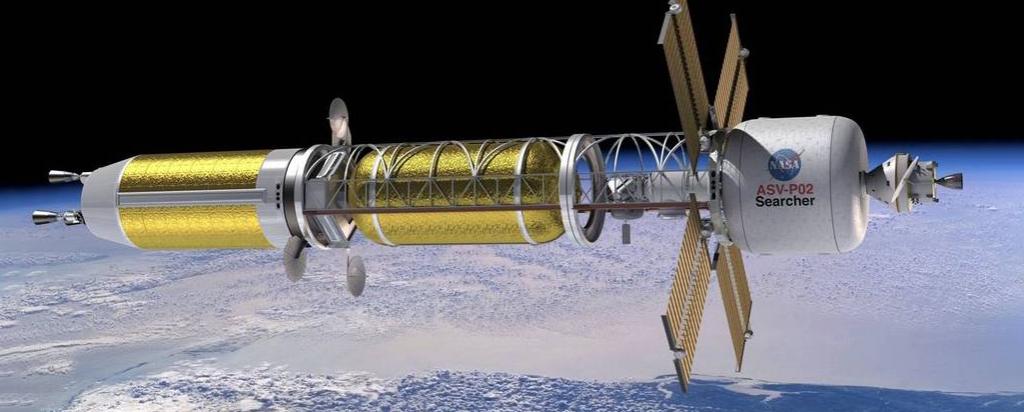
Nuclear propulsion systems
Attempts have been made to develop nuclear propulsion systems for air, land, sea, and space domains; however, only the space and sea environments proved to be practical for the deployment of nuclear propulsion systems.
This is mostly due to the safety, security, and heavy shielding required to contain high amounts of radiation generated during the fission process, which make the practicality of nuclear-powered aircraft and land vehicles challenging.
Several successful nuclear propulsion systems have been developed for space and naval domains, which demonstrate significant advantages over any other currently available source of propulsion energy.
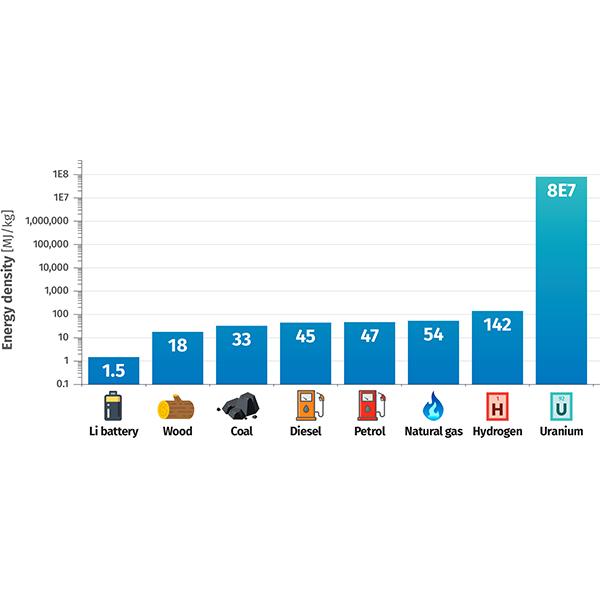
Energy density of nuclear fuel
Energy density of nuclear fuel is vastly higher than the energy density of all other fuels in use. For example, coal is 33MJ/kg, petrol is 47MJ/kg, and hydrogen is 142MJ/kg, whilst U-235 is 8x107MJ/kg. The reason is the large difference between the electronic bonds and the nuclear bonds and that makes nuclear fuel very attractive for propulsion systems. The current technologies utilise the fission process (splitting of atoms), which generate large amounts of thermal energy, utilised directly or indirectly for propulsion.
Applications
Space
The use of nuclear propulsion is the only practical technology, which allows the development of deep-space missions to the outer parts of the solar system, Photovoltaic solar arrays are unsuitable for this environment. Several different concepts of reactor-powered engines exist; however, the focus is on two types of nuclear propulsion systems: (i) Nuclear Electric Propulsion, and (ii) Nuclear Thermal Propulsion.
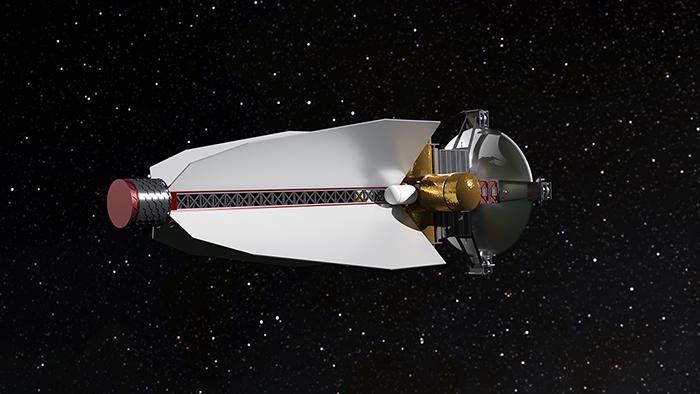
Spear Probe is NASA's ultra-lightweight nuclear electric propulsion probe
Nuclear Electric Propulsion converts thermal energy generated by a nuclear reactor into electricity, which is then used to ionise (positively charge) an inert gas propellant (Xenon, Krypton). In the final step, an ionized propellant is accelerated out of the thruster by an electro-magnetic field creating a low amount of thrust-pushing the spacecraft forward.
These low-thrust ion thrusters can accelerate spacecraft for extended periods. They outperform high-thrust short-burst chemical rocket engines. Ion thrusters are already deployed on satellites, and space probes; however, they are typically powered by electricity-producing solar arrays. As the use of photovoltaic solar cells becomes inefficient further from the sun, nuclear-powered thrusters are the only reliable option for deep-space missions.
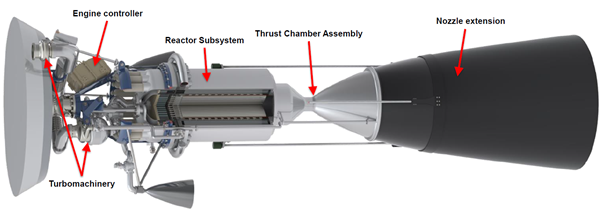
Schematic of a nuclear thermal propulsion system for applications in space
Nuclear Thermal Propulsion directly utilises the thermal energy generated by nuclear fission in a nuclear reactor. This is unlike Nuclear Electric Propulsion, which uses thermal energy to generate electricity.
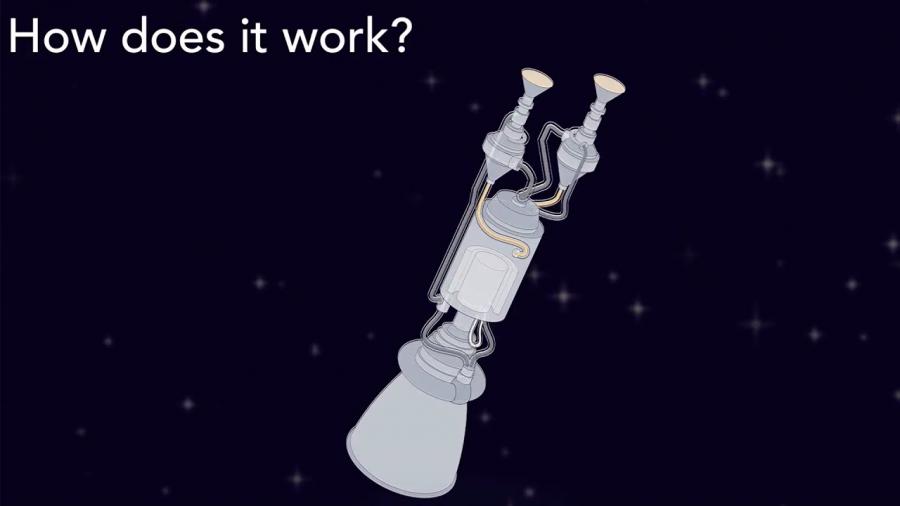
This video from the US Department of Energy explains nuclear thermal propulsion for space applications
Nuclear Thermal Propulsion uses a liquid propellant such as liquefied hydrogen, which is pumped through the reactor core, where it rapidly heats up and expands to a gas, which is then forced out of the rocket nozzle creating high thrust and accelerating the spacecraft forward. Nuclear thermal propulsion is about twice as efficient as chemical rockets and can shorten travel times while delivering greater payloads. For example, a trip to Mars can be reduced by a quarter. Reducing the flight time is particularly important for crewed missions, as short travel times would reduce the flight crew’s exposure to harmful cosmic radiation.
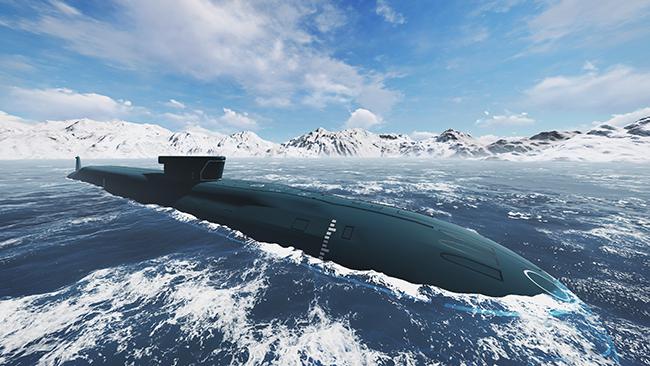
Marine
The most successful deployment of nuclear propulsion to date is in defence navy applications for nuclear-powered aircraft carriers, and submarines.
Nuclear propulsion provides several advantages, such as no need for refuelling for the life cycle of a vessel, higher speeds, and no greenhouse emissions. Furthermore, these systems are safe, reliable and widely spread as they are based on the so-called Pressurized Water Reactor (PWR) design.
The PWR is the most common reactor design for civilian electricity-generating power reactors. This reactor design uses light water as a neutron moderator that slows down fast neutrons in order to sustain a nuclear chain reaction in the reactor core. Light water under high pressure is also used as an energy transfer medium transferring the heat released in the fission process from the reactor core to a steam generator.
In the steam generator, the heat is transferred to low-pressure water of a secondary system where steam is generated to drive turbines, which spin electric generators producing electricity. In principle, marine nuclear propulsion systems are very straightforward in harvesting heat generated by the fission process.