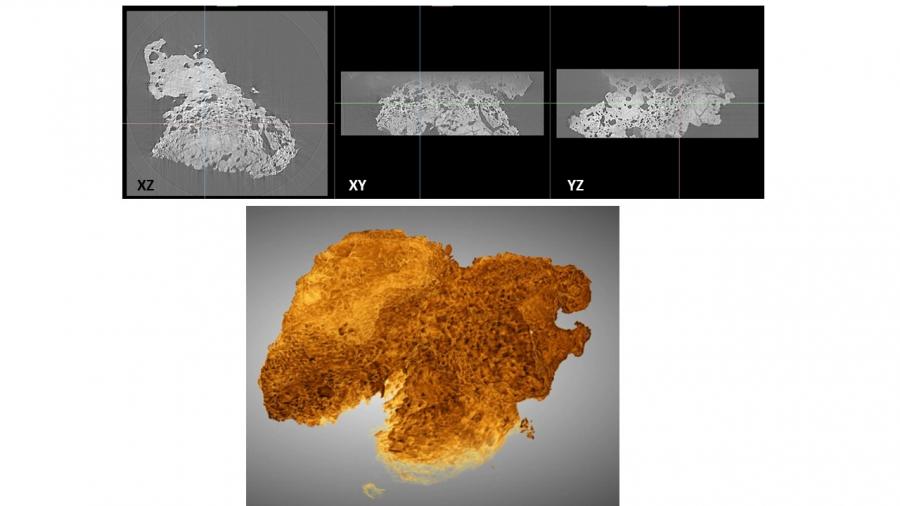
Micro-Computed Tomography beamline (MCT)
The Micro-Computed Tomography (MCT) beamline uses monochromatic, pink, and white X-ray beams to provide detailed three-dimensional (3D) structures at high spatial resolution (down to 200 nm) for a wide variety of technologically-important samples, materials and biological systems.
Capability summary and techniques
The MCT beamline complements the existing X-ray imaging and tomography capabilities provided by the Imaging and Medical Beamline (IMBL), and targets applications requiring higher (sub-micron) spatial resolution and involving smaller samples. MCT is a bending-magnet beamline, operating with X-rays in the energy range of 8 to 40 keV. Monochromatic X-rays is selected using a double-multilayer monochromator (DMM). The DMM operates with a relatively wide energy bandpass (ΔE/E~3%) in order to increase the integrated flux at the sample position. The DMM can also be removed from the X-ray beam path, enabling experiments with a high flux, filtered white beam, for studies that require high-speed data acquisition. The photon-delivery system (PDS) has a single-(vertical) bounce mirror (VBM), capable of suppressing harmonic contamination in low-energy monochromatic beams and providing the means to shape the spectrum of filtered white beams on the high-energy side. The beamline is greatly benefit from novel phase-contrast modalities (such as propagation-based, grating-based and speckle) in addition to conventional absorption-contrast computed tomography. The beamline will be equipped with a robotic stage for high-throughput automated sample exchange. A higher-resolution (nano-CT) configuration based on the use of a Fresnel zone plate system will also be available.
The wide variety of experimental configurations, designed in response to the requirements of a diverse user community can be achieved using three different X-ray imaging detector systems. These will address key issues such as spatial resolution, contrast, field-of-view, frame rate and white-beam compatibility.
In addition to standard “step-and-shoot” and “on-the-fly” modalities for absorption- and phase-contrast X-ray tomography, MCT will provide capabilities for tomosynthesis, laminography, and helical scanning. It is envisaged that absorption-contrast and propagation-based phase-contrast imaging will be “workhorse” techniques, however the other phase-contrast modalities will be made available for more specialist requirements, such as dark-field imaging. Rapid two-dimensional imaging of dynamic processes will also be possible, including via various forms of triggering.
Streamlined data collection, processing, reconstruction, segmentation, rendering and analysis protocols and pipelines will be available via innovative software (including specialised algorithms such as for phase retrieval) and high-performance computing infrastructure.
Scientific applications
Anticipated application areas for non-destructive 3D sample characterisation include biomedical and health science, food science, geosciences, materials science, and palaeontology. A number of sample environmental stages, such as for high temperature and the application of loads, are planned in collaboration with key groups within the user community.
The MCT beamline has been designed in response to the needs of the user community (including industry) for non-destructive structural characterisation of materials down to the sub-micron spatial scale. Examples of applications include:
- Studies of failure mechanisms in 3D-printed (additive manufacturing) samples and various alloy systems. In the case of additive manufacturing samples, it is possible to obtain valuable information on surface morphology as a function of the build direction and the size and complexity of the design;
- Quantifying micro-porosity (e.g. pore-size distribution, shape, connectivity and tortuosity) in rocks and shales for the petroleum industry. The use of gaseous contrast media with K-absorption edges accessible to the MCT beamline shows considerable promise as a non-destructive characterisation and high-resolution tool to study gas-diffusion dynamics and important mechanisms at play such as adsorption.
- Furthering understanding of structural properties for coal and coke can also be obtained. For example, changes to the structure of coke as a result of production methodology (such as blast-furnace parameters) can be studied. An example of results from a coal sample is shown below:
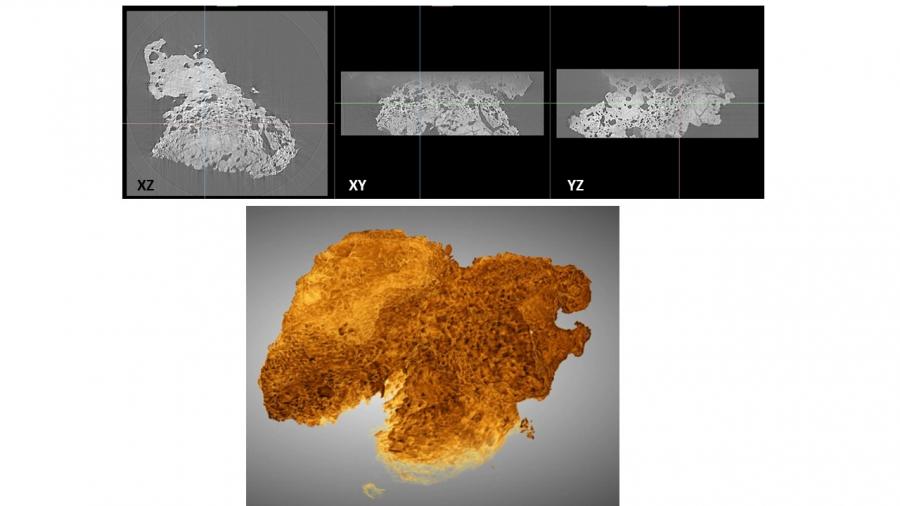
Demonstration of X-ray micro-tomography of coal fragment in small Eppendorf tube (OD ~ 10mm) acquired during the hot commissioning phase of MCT Beamline with the following detail: White beam with heavy in vacuo filtration (0.2 mm diamond, 5 mm Al & 0.5 mm Cu); Optique Peter white-beam detector system with 1X objective; sample is mounted on general-purpose air-bearing CT stage on table B1 located at ~21.5 m from source; 30 flat fields & 30 dark currents are collected at start & finish, 1801 images collected over 180 deg in 0.1 deg steps – “step-&-shoot” mode – exposure time per frame 20 msec – total collection time ~100 min (less than 40 sec exposure time for all frames); total data size ~20 GB – file format TIFF.
- In situ investigation of bone response under load conditions and tensile testing. The use of phase-contrast techniques can greatly enhance the information content in X-ray images of bone;
- Phase contrast can also be especially beneficial in elucidating the presence of fine, low-Z materials in matrix structures, particularly when relatively well-defined edge structure is present - see the example shown below;
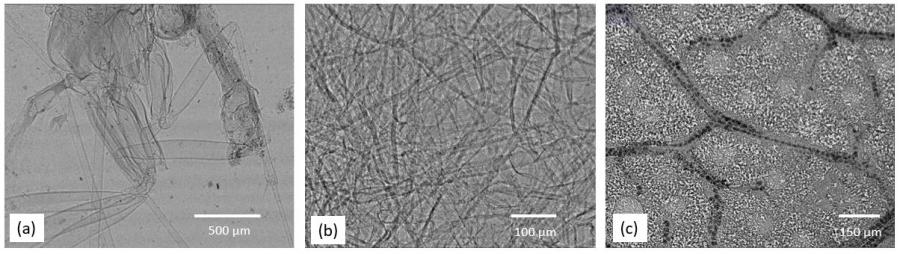
X-ray phase-contrast images (11 keV monochromatic with 5X objective lens) collected at MCT beamline: (a) an insect, (b). fibrous paper (c). an eucalyptus leaf. Phase contrast is clearly present in these images (manifesting itself as black-white fringes enhancing edge structure in particular).
- Characterisation of materials undergoing structural phase transitions in response to temperature or pressure;
- Observation of the evolution and dynamics for metallic foams;
- Soft- and hard-tissue studies using relatively low-energy X-rays to enhance contrast for subtle features;
- Pyrolysis studies for biomass systems;
- High-resolution investigation of various fibre and yarn systems;
- Non-destructive characterisation of small fossils, such as those encapsulated in amber.
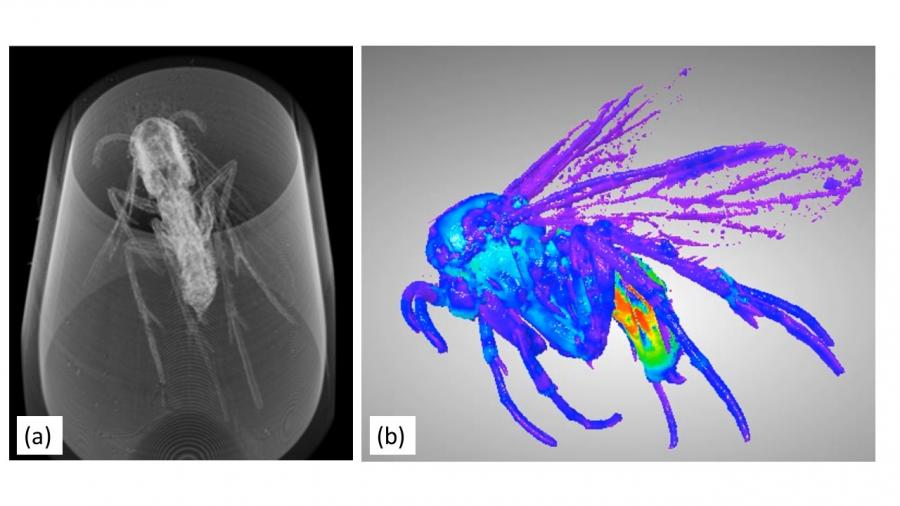
Phase-retrieved tomography result for an insect inside small Eppendorf tube (ID ~ 5mm). (a). 3D rendering of the reconstruction data, (b). 3D colour segmentation using Dragonfly software. The data was collected using 12.7 keV monochromatic (Ru/C stripes), in vacuo filtration – 0.2 mm diamond, with 1X objective lens in the imaging detector, 1.5 m sample to the detector distance, 1801 images collected over 180 deg in 0.1 deg steps, 30 flat fields & 30 dark currents, “step-&-shoot” mode – exposure time per frame 40 msec – total collection time ~100 min – total data size ~19 GB – file format TIFF. TIE-Home phase retrieval was applied on the projection images before 3D reconstruction.
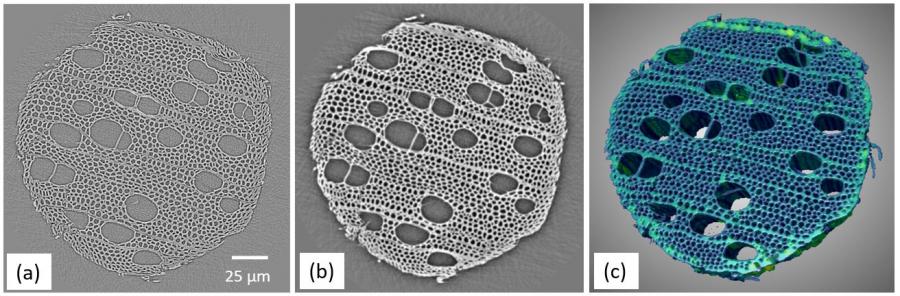
Slice reconstruction of wood structure (toothpick) without TIE-Hom phase retrieval in (a) and with TIE-Hom phase retrieval in (b) with the 3D visualization of the (b) in (c). Data was taken using white beam, in vacuo filtration – 0.2 mm diamond, 5 mm Al, 0.1 mm Cu, using 20X objective lens, 1201 number of projections, 100 flats & 100 darks, sample-detector distance of 160 mm, exposure time of 0.7 s.
Technical Information and Specifications
The MCT beamline comprises three hutches centred at approximately 15, 24 and 31 m from the bending-magnet source point; all three are white-beam compatible. The first hutch (the first optical enclosure) houses the photon-delivery system, including mask, slits, collimator, DMM, VBM, diagnostics and shutters. The X-ray beam travels in vacuum until it passes through the Be window at the entrance to the second hutch, approximately 20 m from the source. The second and third hutches constitute the end stations for conducting user experiments. When the experiments are in the second hutch, a beam stop at the downstream end provides for safe and open access to the third hutch. When the experiments are in the third hutch, the second hutch is searched and secured.
Each of the experimental end stations house two large, stable optical tables to support experimental equipment. These tables can be adjusted in height and (for the first end station) tilt, so that a fixed offset is maintained between the table surface and the X-ray beam for any configuration of the DMM and VBM in the photon-delivery system.
The first experimental hutch houses the sample-exchange robot that is fixed on the first experimental table, and will operate with the primary CT sample stage. Standard, absorption-contrast and propagation-based phase-contrast X-ray imaging and tomography will be routinely performed on this table; however it will also be possible to use the second experimental hutch in cases where a larger field-of-view is required.
The first optical table set-up in the first experimental end station.
The general-purpose CT stage, which can be moved as required.
Several example for sample mounting.
Fresnel zone plate optics will be housed on the second table in the first experimental hutch for high-resolution (“nano-CT”) studies down to 200 nm spatial resolution. For these studies, it is necessary for such experiments to straddle both end stations, with the nano-CT detector being located in the second experimental hutch. The more specialised phase-contrast modalities such as grating-based and speckle imaging will also be set up in the second experimental hutch using the third and fourth experimental tables. Configurable evacuated flight tubes will be available in order to avoid attenuation and scattering of the X-ray beam in appreciable air paths, especially at lower energies.
Detectors
There are two different types of detectors available in MCT Beamline. They are CMOS- & CCD-based with scintillators & specialised magnifying optics.
(a). White beam detector with three objective magnification lenses. (b). Mono-beam detector with three objective magnification lenses.
Technical Specifications
View MCT Technical Specifications
Source | Australian Synchrotron bending magnet (1.3 T); critical energy 7.95 keV |
Hutches | 9-BM1-A (~15 m); 9-BM1-B (~24 m); 9-BM1-C (~31 m) |
Energy range | 8 – 40 keV (also filtered-white & pink beams available) |
Integrated flux | ~ 5 x 1016 photons/sec unfiltered after mask* (~ 50 W) |
PDS filters | 5 paddles, each with 4 filters - incl. C (graphite), C(diamond), Al, Cu, Zr, Rh & Sn |
Monochromator (DMM) | 3 multilayer stripes: Ru/C & W/B4C with ∆E/E ~ 3% for 8 – 23 keV & 21 – 40 keV; V/B4C with ∆E/E ~ 0.5% for 8 – 25 keV |
Mirror (VBM) | Single-bounce vertical, bendable (concave & convex); Rh & Pt stripes |
Optical tables | 3 m x 1 m (2) & 2 m x 1 m (2); ~300 mm vertical & 15 mrad tilt adjust.; 100 µm & 0.1 mrad resolution |
PDS slits | 3 sets, low-scatter, white-beam; range 50 mm (H) x 80 mm (V) |
Beam size | Max. *2.0 mrad (H) x 0.3 mrad (V) for filtered-white & pink beams - 44 mm (H) x 6.6 mm (V) for sample positioned at 22 m, 64 mm (H) x 9.6 mm (V) at 32 m; max. 1.6 mrad (H) x 0.3 mrad (V) for monochromatic beam |
Sample handling & environments | Robotic stage for automated sample exchange; environments yet to be finalised - likely to start with high-temperature & load cell |
Detectors | CMOS- & CCD-based with scintillators & specialised magnifying optics |
Beamline Status
View MCT Beamline Status
1st July 2017 | Project started | |
September 2017 | Capital Investment Case endorsed & approved by ANSTO | |
20th October 2017 | First Beamline Advisory Panel (BAP) meeting convened | |
June 2018 | Conceptual Design Report endorsed | |
27th March 2019 | Contract for DMM awarded to Axilon | |
21st May 2019 | Contract for PDS (excl. DMM) awarded to Axilon | |
June 2019 | Technical Design Report completed | |
25th June 2019 | Contract for hutches awarded to Caratelli | |
November 2019 | Lead Scientist & Lead Engineer visit TOMCAT beamline (SLS) & Axilon | |
12th December 2019 | Approval of Final Design Review report for PDS (incl. DMM) | |
January 2020 | 2nd Beamline Scientist joins MCT Team | |
* Plus delay to be determined following resolution of covid-19 related restrictions | ||
23 November 2021 | MCT first light | |
14 July 2022 | First phase contrast images |
|
4 August 2022 | First computed tomography scanning | |
13 September 2022 | Day 1 | |
6 October 2022 | First User | |
10 February 2023 | ARPANSA approval | |
12 September 2023 | Day 365 |
Beamline layout
The Micro-Computed Tomography beamline layout.
Find out more on the Australian Synchrotron User WikiView the Wiki
Staff
- Dr Prithi Tissa - Project Manager
- Dr Andrew Stevenson - Lead Scientist
- Dr Benedicta Arhatari - Beamline Scientist
- Mr Adam Walsh - Lead Engineer
- Mr Tom Fiala - Senior Controls Engineer
Dr Darren Thompson - Snr Scientific Software Engineer
Dr Gary Ruben - Snr Scientific Software Engineer
Mrs Tingting Feng - Scientific Software Engineer
- Miss Emily Griffin - Mechanical CAD Designer
Micro-Computed Tomography Beamline Advisory Panel
- Dr Sherry Mayo (Chair) - CSIRO
- Dr Kaye Morgan - Monash Univ.
- Dr Andrew Rider - DST Group
- Dr Ian Schipper - Vic. Univ. Wellington, New Zealand
- Prof Adrian Sheppard - Australian National Univ.
- Prof. Marco Stampanoni - TOMCAT, SLS, Switzerland